Biochar – the key to better Cannabis growth
If you want healthier Cannabis plants and abundant yields, improve your soil health with activated biochar. Its effects last a long time if you apply this organic matter properly. Remember that excessive use can lead to detrimental results.
We mix our own Living Soil. Here is an example of a 2500 Plant Grow where Biochar is being used. Some Grow Containers we re-use. This can be done by adding Tea to replace previously used or missing Micro/Macro Nutrients.
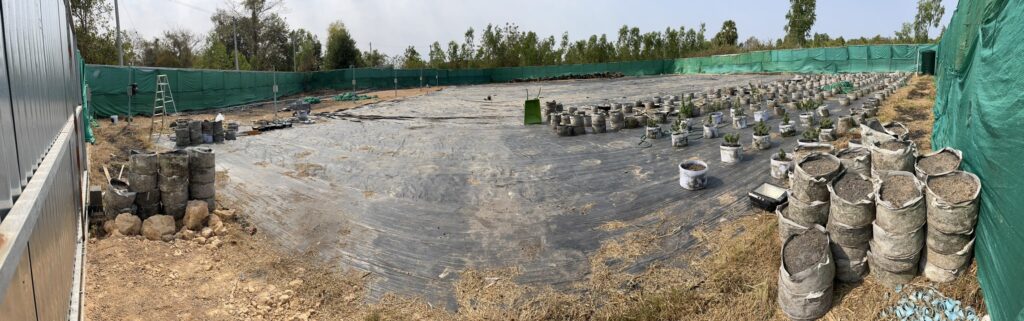
Biochar is used as a Sustainable Alternative and Green Absorbent for the Remediation of Noxious Pollutants. Along with being an excellent soil amendment.
Mycorrhizal Fungi, a Symbiotic Parasitic Rhyzone thing we use takes up to 3 mos to fully Mature/Grow. These containers are full of rhizo-spheric microbiomes and Fungi. Save some money for Whiskey too!
Biochar is an excellent soil amendment providing housing for Bacteria and Fungi like Mycorrhiza and also can be used to absorb pollutants. Just a couple aspects of this Amazing Substance and it’s use in our Lives and our endeavor to Clean up our planet.
Yea, Just like Hemp. Biochar is an answer to renewing the Earth’s Soil!
Compared with other Soil Amendments, Biochar is considered as an environmentally friendly, sustainable, and cost-effective substance for soil improvement, waste management, climate protection, wastewater treatment, etc.
The special properties of biochar such as porosity, surface area, surface charge, and functional groups can be easily modified by various chemical methods, resulting in improved properties and different applications.
Due to the wide range of applications of biochar, the conversion of biomass into biochar has increased. To produce biochar, the raw material is collected and subjected to thermal decomposition under various conditions. Different processes are used to convert biomass (raw material) into biochar depending on the temperature range, conditions, and residence time, namely pyrolysis, hydrothermal carbonization, torrefaction, and gasification.
All processes produce biochar (solid) as the main product, as well as bio-oil (liquid) and biogas (gaseous) in larger or smaller quantities. The percentage of solid, liquid, and gaseous products varies from method to method. The method is selected depending on the biomass in order to obtain the maximum yield of biochar.
The properties of the feedstock and the method used for biochar production have a great influence on the physical and chemical properties of biochar, so it is crucial to understand the heating rate, residence time, and decomposition temperature of the different production methods. The methods used to produce biochar are explained in detail below:
How is biochar made?
How is biochar made? Heating the Biomass with little or no Oxygen present. A process called Pyrolysis. Use only organic materials as feedstock. Do not use items with contaminants can produce char that’s potentially harmful to your Marijuana plants.
The temperatures you use in making biochar will also determine the resulting organic matter type. The variety created by low heat is better for immediate outcomes, while those produced in high temps have a longer-term effect on your soil.
How to make biochar at home?
If you’re interested in how to make it at home, consider these ideas. They don’t require expensive equipment and are simple to execute.
Trench method
Start by digging a trench in your garden. Some users of this option create a cone-shaped pit with the upper portion wider than the bottom.
The next step in biochar production is to put some brush in and light it. Add more biomass as the fire rises. Observe the color of the smoke to know the progress. At the earlier stage, you’ll notice white fumes caused by water vapor.
As the sugars and resins burn when making biochar at home, your fire will emit yellow smoke. Wait till it turns grayish-blue, then smother the flames with about an inch of dirt or sand to reduce the oxygen supply. Leave it to smolder until you get chunks of charcoal. Put out the fire.
Barrel method
An alternative biochar production method is to burn wood pieces in metal drums. Many producers use two barrels, one smaller in size, that you insert into the larger container.
Place the material you want to turn into biochar into the inner drum and insert wood around it that you will burn. The heat will convert the matter in the smaller vessel into lumps of carbon. Cover the large barrel to reduce the oxygen supply. This is a Great way to make Bio Char and also Carbonized Rice Hulls.
Pryolysis
Exposing the feedstock to a high temperature (250–900 °C) in the presence of limited or no oxygen is called pyrolysis. Pyrolysis has been reported to be the preferred method among all biochar production processes because it can be used for a wide range of biomass. During pyrolysis, the components present in biomass such as cellulose, hemicellulose, and lignin are depolymerized, fragmented, and cross linked.
This leads to the formation of products in different phases such as solid, liquid, and gas. Pyrolysis conditions such as temperature, residence time, and type of biomass play an important role in biochar yield. Pyrolysis can be divided into slow pyrolysis, fast/flash pyrolysis, and microwave pyrolysis depending on the temperature and residence time.
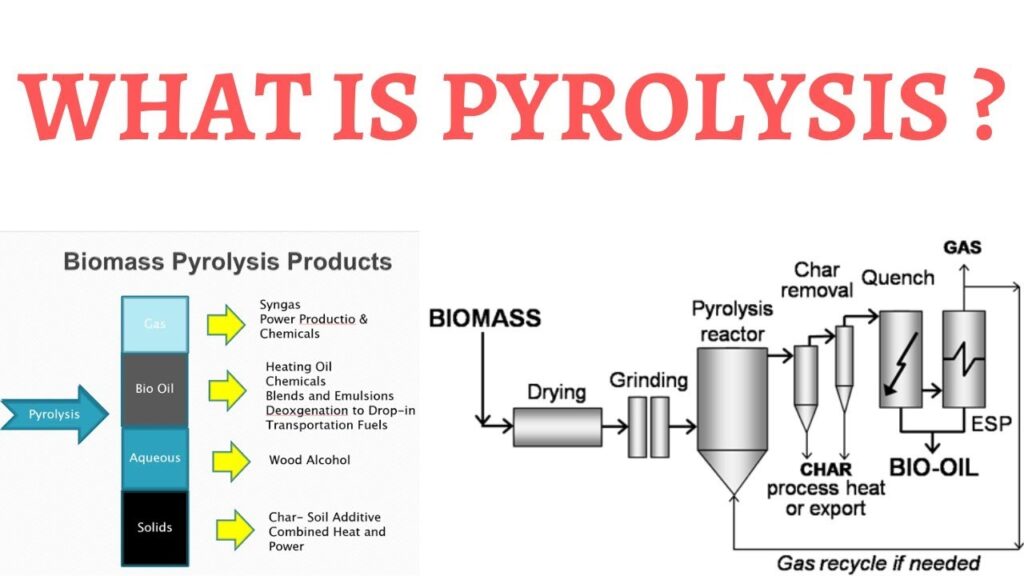
Slow Pyrolysis
In this process, the biomass undergoes pyrolysis at 300–500 °C with a longer residence time of several minutes to days. Slow pyrolysis is considered the most effective because it yields a maximum of solid products (biochar) and a lower level of gaseous (biogas) and liquid products (bio-oil). In general, as the temperature increases, the yield of biochar decreases and the amount of biogas decreases.
Since slow pyrolysis involves a slower heating rate and longer residence time, maximum biochar yield is obtained. According to one study, slow pyrolysis achieves a biochar yield of 35.0% from dry biomass. This is the main reason why slow pyrolysis is the most suitable method for biochar production among all pyrolysis processes.
Fast pyrolysis
Fast pyrolysis is a thermochemical process for liquefying biomass into bio-oil, which is highly useful for energy production. It is carried out at a temperature above 500 °C to 700 °C. This type of pyrolysis involves faster heating rates (>10–10,000 °C/min) and shorter residence times in seconds. As mentioned earlier, the yield of biochar from this process is comparatively low compared with slow pyrolysis due to the increase in temperature and reduction in residence time. Instead, a maximum amount of bio-oil is obtained at the end of the fast pyrolysis.
The type of pyrolysis process also affects the properties of biochar produced. A higher pyrolysis temperature results in biochar with a larger surface area, higher pH and volatiles, but lower surface functional group and cation exchange capacity (CEC).
Microwave pyrolysis
Microwave pyrolysis is the decomposition of biomass by energetic microwave radiation. Unlike conventional pyrolysis, in microwave pyrolysis the temperature at the center of the feedstock is higher than at the surface. This results in efficient heat transfer and shorter reaction time compared with other thermochemical methods.
The process is cost effective as microwave pyrolysis provides rapid and uniform heating, i.e., energy intensive and steps such as preheating and dehumidification is not essentially required in this process. However, microwave pyrolysis yields a very small amount of bio-oil compared with other conventional processes.
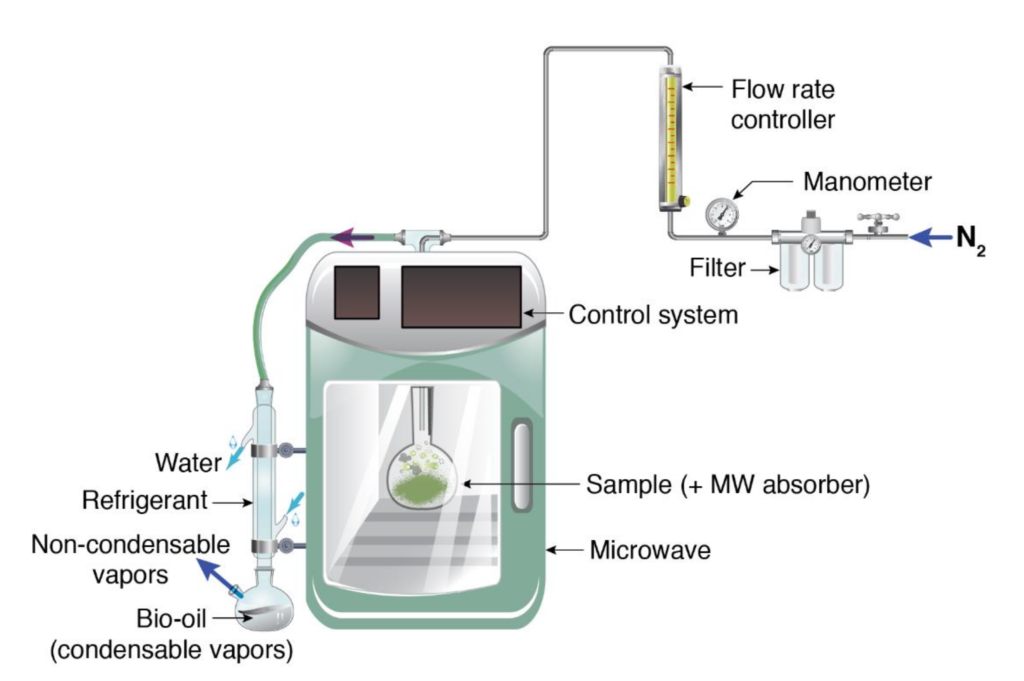
Hydrothermal Carbonization
In hydrothermal carbonization, also known as “wet pyrolysis”, the biomass is surrounded by water during the reaction. In this process, the reaction temperature is applied in a range of 180–230 °C under low pressure in a closed system. In the HTC method, the pressure and temperature are maintained in such a way that the water remains in a liquid state throughout the process.
Further, the advantages of HTC includes energy efficient production of biochar in high yield, i.e., low temperature requirement (about 180 °C). It involves a series of reactions such as hydrolysis, condensation, decarboxylation, and dehydration. The HTC method for the production of biochar is attracting more and more attention because it offers the advantage of using wet biomass and does not require an additional drying step.
To distinguish the biochar obtained by pyrolysis and HTC, the final product of HTC is called “Hydrochar”. The Hydrochar formed has more oxygen-containing functional groups, which makes it more favorable for adsorption of heavy metals from aqueous media. Reports indicate that Hydrochar has more oxygen-containing groups and higher CEC value than the biochar produced by pyrolysis, which is an important factor for the use of biochar as an absorbent. The easy biodegradability of Hydro-char limits its application for carbon sequestration.
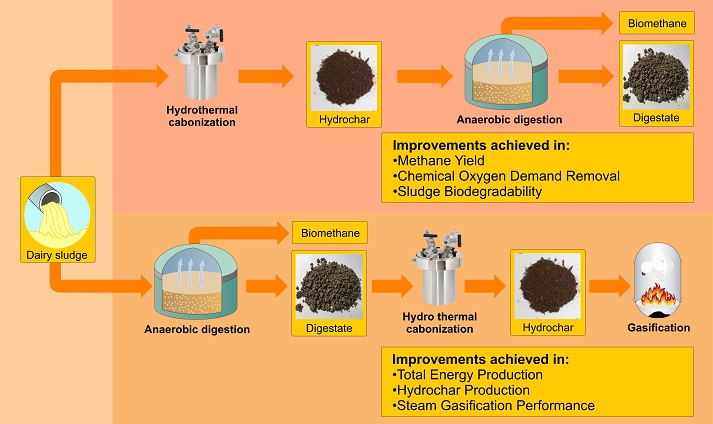
Torrefaction
Torrefaction is one of the new techniques for biochar production from biomass. In this process, raw material was heated for the residence time of 15–60 min within the temperature range 200–300 °C in an inert atmosphere. Since, the operating temperature is lower than that of pyrolysis, it is also called “mild” pyrolysis. In this process, biomass depolymerization occurs, the extent of which depends on the reaction time and temperature. Biochar is not the main product of torrefaction, but is suitable for adsorption of pollutants. The biochar produced by this process exhibits hydrophobic properties because the hydroxyl groups present on the surface of the biochar are removed during this process.
In addition, the biochar obtained from torrefaction has better ignitability, grindability, high calorific value, carbon content, energy yield, and lower moisture content, which makes it more suitable for use as a fuel.
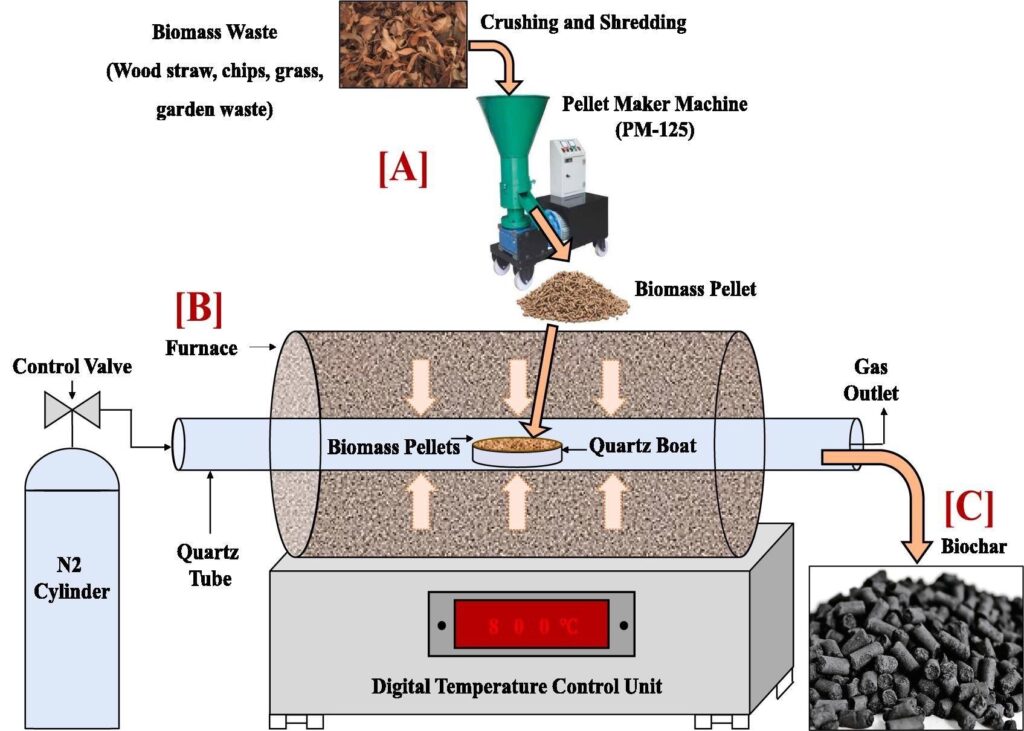
Gasification
Gasification of biomass is shown as another alternative method for the synthesis of biochar. The biochar was synthesized in an oxidizing environment (single or mixture of gases) at a temperature of ~700°. Further, the partial combustion of the feedstock under oxidizing atmosphere produces syngas, a mixture of H2, CO, CO2, and CH4, which is used as fuel. The reaction temperature is the key factor affecting the production of syngas.
Gasification produces the highest percentage of gaseous product and very little biochar compared with the other processes. The biochar obtained by this process is more stable and resistant to chemical oxidation, and has a smaller particle size. Biochar contains minerals such as N, K, P, and Ca which increases the soil fertility and enhances the growth of plants.
Converting biomass into biochar is an effective strategy for waste management and environmental protection. Decomposition of organic materials releases harmful gases such as carbon dioxide and methane into the atmosphere, which contributes to global warming. Through biochar production, solid waste issues of biomass can be managed and it can also prevent the production of greenhouse gases, thereby contributing towards the reduction in GHGs.
Moreover, it reduces the cost associated with the solid waste disposal. The excellent performance of biochar as an adsorbent can be attributed to its characteristic properties. Some of the specific properties of biochar are, it’s porous nature, high carbon content, high specific surface area (SSA), hydrophobic nature, and ease of functionalization.
Further, the composition of biochar in terms of carbon, nitrogen, sulfur, and oxygen affects the chemical properties of biochar. The properties of biochar depend on several factors such as composition of the raw material, preparation method, and pyrolysis temperature. Thus, it is important to understand how the reaction conditions influence the property of biochar.
Therefore, in order to develop biochar with desired properties or to treat the analyte of interest, it is inevitable to have a thorough knowledge of all the factors affecting its properties. For example using Biochar in your Grow. The mineral content of biochar varies greatly depending on the feedstock used. Poultry litter biochar has a higher mineral content than all other types of biochar produced. The pH of the resulting biochar also varied depending on the feedstock used. A very important aspect in Growing anything is the PH.
As the temperature increases, porosity and particle size of the biochar also increases. The smaller particles have larger surface area and better CEC value. The CEC value also depends on the type of raw material used for preparation of biochar. This “Porosity provides “Housing” for bacteria and Fungi (Mycorrhiza)
In addition to the raw material, the decomposition temperature in the production of biochar is also a key factor affecting the adsorption of heavy metals on biochar. It is observed that as the pyrolysis temperature increases, the functional group containing oxygen decreases thereby altering the absorption efficiency of the biochar. Moreover, the pH of the solution also plays a significant role in deciphering the absorption capacity of the absorbent; it can increase or decrease the absorption capacity based on the model metal impurity.
Biochar possesses excellent absorption capacity towards the remediation of several noxious pollutants whether it is organic or inorganic. Thus, based on the remediation activity it is the need of the hour to document the different absorption mechanisms under one paper for a better understanding of the readers and researchers across the globe.
These absorption mechanisms not only help us to gain insights but also play a significant role in policy making for enhancing the application of biochar from lab to pilot scale through increasing their removal efficiency and large-scale production. There are certain interactions and forces that act between the target molecule and the surface of biochar.
The interaction between adsorbent and adsorbate majorly depends on the factors such as nature of pollutants, pore nature, pore volume, specific surface area, hydrophobic nature, and surface functionalization, etc.
The different adsorption mechanism involved during biochar–pollutants interaction. Based on the interaction with different organic and inorganic pollutants, they are classified as coagulation, precipitation, ion exchange, electrostatic interaction, hydrophobic interaction, pore-filling interaction, and hydrogen bond formation.
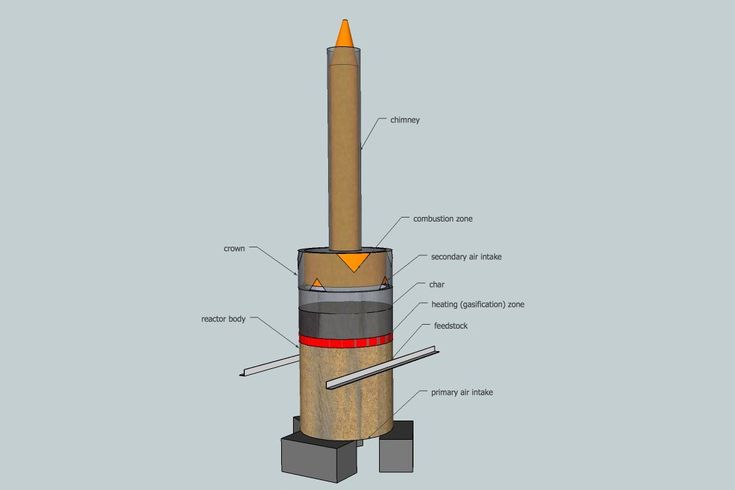
Complexation
This mechanism involves transition metal to ligand ( a ligand is a substance that forms a complex with a biomolecule to serve a biological purpose.) Interaction thereby leading to the formation of complex chelate compounds. The oxygen-containing groups at the surface sites interacts with the free orbital of transition metals to form complexes. Further, due to oxidation of biochar surface oxygen content over the time also increase thereby enhancing metal complexation.
Precipitation
This is the most prevalently followed mechanism for adsorptive removal of heavy metals ions onto the biochar. The solid precipitates either in the solution or the adsorbent surface were formed under this mechanism. Further, the heavy metals having ionization potential in the range of 2.5–9.5 eV generally absorbed on to the biochar surface following the precipitation mechanism.
Water pollution is increasing due to the different factors such as population growth, urbanization, industrialization, etc., which is a major concern worldwide. Environmental pollutants can cause various diseases, neurological problems, and cancer. The wastes generated by industries are released into the water bodies, atmosphere, and soil, contributing to the pollution of soil, water, and air.
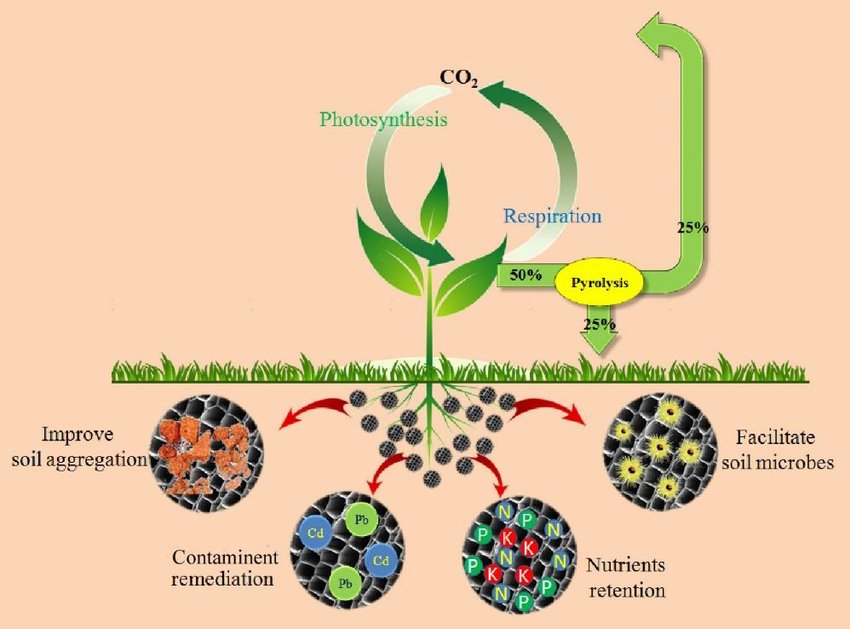
Pollution results in adverse effects on the entire ecosystem, especially on human life. Most pollutants that pose a threat to the environment originate from industry. Industrial wastewater, which consists of organic and inorganic substances, is toxic to humans and must therefore be treated before it can be released into the environment.
Researchers are continuously striving to reduce pollutants. Generating sustainable and green energy sources to meet the needs of today’s world is one such initiative. In recent years, researchers have also made efforts to find a sustainable, environmentally friendly solution to the pressing problem of waste treatment. Methods such as advanced oxidation processes, absorption, ion exchange, reverse osmosis, ozonation, interfacial solar evaporation, coagulation, and precipitation are used to treat various pollutants.
All reported methods (except absorption) are either associated with high operating costs or are inherently complex; therefore, these methods are not economically viable.
The term “biochar” was first coined by Peter Read in 2015. Biochar is considered a promising candidate because it is a sustainable, cost-effective, and biowaste-based adsorbent along with being an excellent Soil Additive/Amendment.
It is a pyrolytic or anaerobic, thermally treated, stable, and carbon-rich substance obtained from organic material as raw material. Waste materials containing lignin or non-lignin from agriculture, aquaculture, wood, and fiber processing industries, etc., are primarily used for the production of biochar.
Specific properties of biochar include hydrophobicity, long-term stability, chemical composition, large surface area, high porosity, etc. This makes it a potential candidate for multiple applications such as harmful pollutant removal, soil amendment, carbon sequestration, greenhouse gas adsorption, etc.
In addition, the surface properties of biochar can also be adjusted by various modification processes such as acid/alkali treatment, application of other materials to the surface, ball milling, acetylation, etc.
Moreover, biochar has a more environmentally friendly aspect or offers an alternative for environmental management in soil improvement, (I use Biochar in all of my Grows. THC or Hemp) waste management, energy production, and climate change mitigation. Below is a close up of Bio Chars’ Surface.
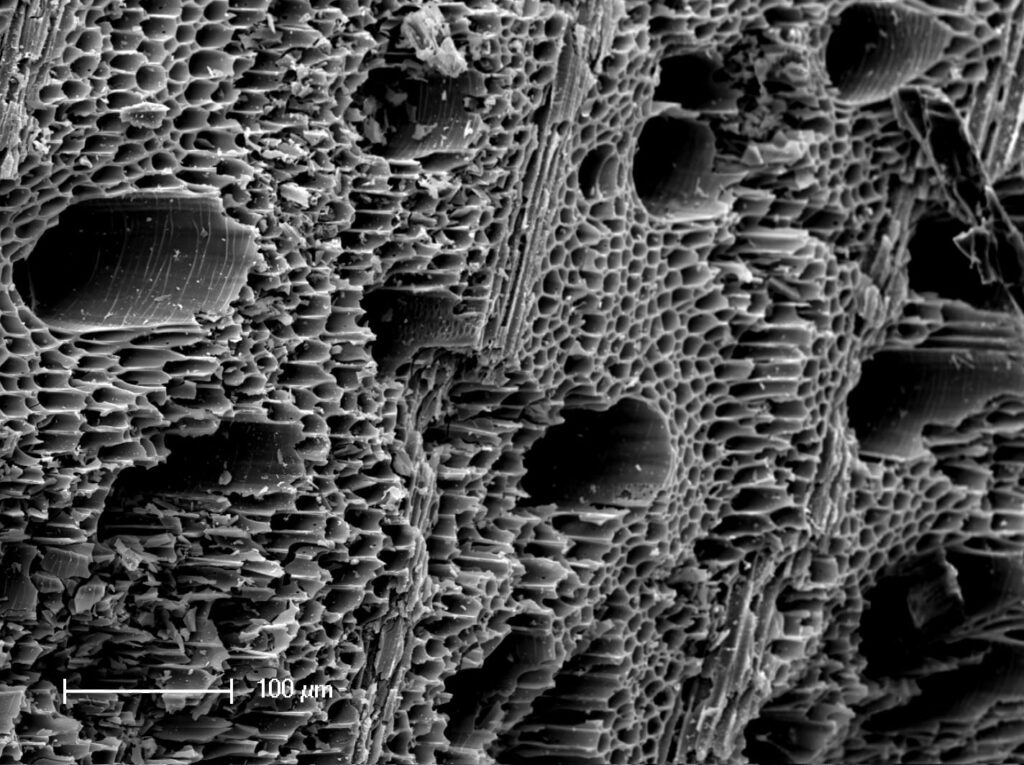
Given the wide acceptance and recent advances in the field of biochar properties and various synthetic routes of biochar adds to the Correlation with Sustainable Development Goals (SDGs).
Ion Exchange
This involves the exchange of ions between the solid-liquid interface. The heavy metal ions present in the liquid phase were moved from the liquid phase to the solid phase, i.e., biochar active sites. It is a reversible process between adsorbate and adsorbent. Further, to maintain the electrical neutrality of the aqueous solution, the exchange of ions takes place. Moreover, the removal efficiency of adsorbent in this mechanism majorly depends on two factors, i.e., model pollutant size and type of surface functionalization.
Electrostatic Interaction
This mechanism is based on the attraction and repulsion of charges, which is the essence of ionic bond formation. If the pollutant to be treated is ionic or readily ionizable, the electrostatic interaction begins to dominate. The cationic pollutants can be easily adsorbed on the negatively charged surface of biochar. The predominance of this mechanism depends on the pH of the solution and the zero charge point (ZCP) of biochar.
At a pH level less than PZC, biochar shows positive charge on its surface promoting the absorption of negatively charged pollutants. At pH greater than PZC, biochar shows negative charge on its surface favoring the adsorption of negatively charged pollutants. Electrostatic interaction has also been described as an adsorption mechanism for negatively charged Cr (VI) on the positively charged surface of biochar. When used in Pollution Remediation.
Hydrophobic Interaction
‘Hydrophobic’ means water-repellent or water-phobic and arises from the presence of nonpolar groups (C-H) in a molecule. This mechanism is used for the adsorption of hydrophobic or neutral organic compounds through hydrophobic interactions. The hydrophobic nature of biochar can be explained by a decrease in oxygen, hydrogen, nitrogen, and sulphur content and an increase in carbon content due to carbonization. (Why it Floats)
The increase in pyrolysis temperature during the production of biochar leads to a decrease in the number of polar groups and enhances the hydrophobic nature of biochar. The adsorption of oil on the surface of biochar occurs mainly due to hydrophobic interactions.
Pore-Filling Interaction
The porous nature of the biochar is the key reason behind the pore-filling interaction mechanism. Biochar possess different types of pores and based on the pore dimensions, the pores that are <2 nm in diameter represent micropores, pores that are in the range 2–50 nm in diameter fall under the category of mesopores, while pores >50 nm in diameter represent macropores.
Further, the pore size of the biochar can be controlled by the number of factors which involves synthesis mechanism, pyrolysis temperature, pre- and post-treatment methods and based on the model pollutants size removal efficiency of the adsorbent can be varied during diffusion and absorption. This mechanism also depends on the polarity of the contaminant. To achieve high adsorption by pore-filling, biochar should have a low volatile content.
Hydrogen Bond Formation
Numerous toxic pollutants are absorbed by the formation of hydrogen bonds on the surface of biochar. The formation of hydrogen bonds occurs due to the negatively charged surface of biochar by the presence of -OH groups. Phenolic compounds, pesticides, a very important consideration for Growers of Cannabis here in Thailand for example is if you preparing an old Field that was previously used to grow Sugar Cane. Dyes, etc., form hydrogen bonds with biochar.
Unfortunately the Thai Farmers are “addicted” to synthetic Fertilizers.
The functional groups present either on the surface of biochar or organic pollutant can participate in the formation of hydrogen bond. Groups such as amine (-NH) and hydroxyl (-OH) serve as H-donor. On the other hand, benzene rings, F, N, and O act as H-acceptors for H-bonding to make it more clear in the revised manuscript.
Applications of Biochar
Rapid industrialization and increased population have led to an increase in concentration of noxious pollutants (organic and inorganic) in the environment. The pollutants can be categorized as organic (e.g., dyes, agrochemicals, etc.) and inorganic (heavy metals). Biochar is a powerful, environmentally friendly, and cost-effective means of combating the current pollution crisis.
Due to its versatility, biochar has been widely used to treat various pollutants in recent years. So far, from past few decades biochar is known to be a low-cost material that was used as successful adsorbent for the remediation and abatement of noxious impurities such as organic dyes, heavy metals, oil spillages, pesticides, carbon dioxide, etc.
The exceptional properties of biochar such as large surface area, porosity, long-term stability, etc., are the main factors for its use as an adsorbent. The properties of biochar can be adjusted depending on the desired application and the analyte to be treated. The type of contaminant/impurity (polar/nonpolar, cationic/anionic, hydrophobic/hydrophilic, and organic/inorganic) also affects the efficiency and applicability of biochar. The following are some areas where biochar has contributed to environmental remediation as an adsorbent:
Remediation of Organic Pollutants
Exponential growth in textile industries lead to the disposal of organic impurities such as dyes, phenolic compounds, PAHs, PPCBs, chlorophenols, etc., into the nearby aquatic resources. These pollutants possess negative impact on the environment as well as human health. Due to their potential threat to the environment, it is imperative to treat them before they are released into the environment.
Biochar has the potential to remove hazardous and poorly degradable organics through an absorption mechanism. Absorption of organic pollutants mainly depends on the surface properties of biochar and the type of pollutant. For example, the absorption of dyes on biochar strongly depends on whether the dye is cationic or anionic.
Remediation of Oil
Oil spills have dangerous effects on the marine ecosystem and on the land (soil). The oil absorption capacity of biochar can be mainly related to its hydrophobic/oleophilic nature, which can be estimated by measuring the water contact angle. In addition to hydrophobicity, other factors such as porosity, surface area, etc., also play an important role in determining the oil absorption capacity of biochar.
Biochar with high porosity has higher oil removal capacity because the oil is trapped between the porous arrays. Biomass with a high lignin content results in biochar that is more hydrophobic and amorphous. This type of biochar shows better oil removal capacity.
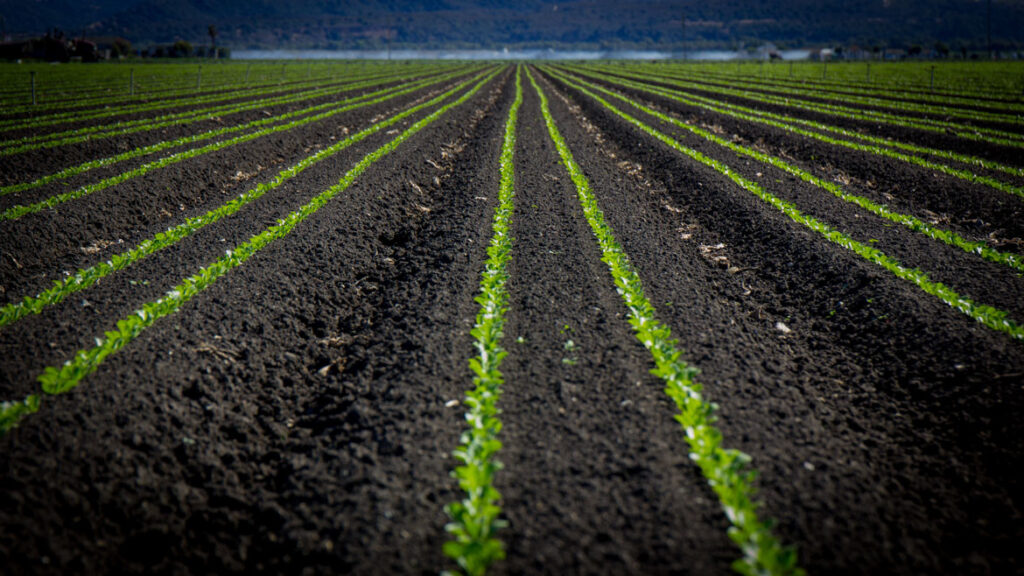
Remediation of Organic Contaminants
Organic molecules such as benzene, penicillin, chlorofluorocarbons, etc., when released into the environment, have adverse effects on the environment and human health. Absorption of organic molecules with biochar is one of the most effective methods to treat toxic molecules. 2,4-dichlorophenol was adsorbed from water using wheat husk-derived biochar. Biochar treated with cerium trichloride was used to treat levofloxacin (drug). Cerium treatment increased the oxygen-containing functional group on the biochar surface, resulting in improved adsorption performance from 37.80 mg/g to 98.33 mg/g.
Remediation of Inorganic Pollutants
Hemp is currently being used to help clean up the Soil around the 1986 Chernobyl Nuclear Plant accident in Russia.
Inorganic pollutants mainly include heavy metals such as lead (Pb), mercury (Hg), cadmium (Cd), zinc (Zn), etc., which enter the environment through industrial wastewater. The presence of these metals, even in very small amounts, can have a significant impact on the environment. Adsorption has proven to be a universal, fast, convenient and effective method for the removal of these hazardous pollutants.
Biochar has a strong affinity for heavy metals due to its surface area, porosity, and the oxygen-containing functional group on its surface. The mechanisms involved in the immobilization of heavy metals are generally ion exchange, precipitation, electrostatic interaction, and complexation. The absorption performance depends on the type of heavy metal (cationic/anionic) and the amount of oxygen-containing functional groups (carboxylate and hydroxyl) on the surface of biochar.
The size of the pores and the surface area of biochar affect the performance of biochar. The feedstock used as raw material for biochar production also affects the heavy metal sorption capacity of biochar. Biochar produced from agricultural and forestry residues improve the sorption capacity for heavy metals. This may be attributed to the numerous oxygen-containing functional groups on the surface of biochar, which provide negatively charged surface sites favorable for adsorption of heavy metals.
The use of animal waste such as poultry litter for biochar production increases the ash and inorganic content of the biochar. This improves the binding of heavy metals to the surface of the biochar. Mineral components such as CO32− and PO43− from the feedstock serve as additional absorption sites for the absorption of heavy metals on biochar.
Engineered Biochar
The main advantage of biochar is that its properties can be adjusted depending on the substance to be treated. The term “engineered biochar” is used for biochar that has been modified or activated using various techniques. In recent years, there has been an increase in the development of EB to expand the applications of biochar.
Modification techniques for biochar production can be divided into chemical, physical, and biological. Techniques such as acid/alkali treatment, impregnation of the surface with other materials, ball milling, acetylation, etc., are used to tune the properties of biochar. These modification techniques can be used before (biomass treatment/specific reaction temperature) or/and after (treatment of pure biochar) the production of biochar.
Schematic representation of different methods used for preparation of engineered biochar and their effect on the properties of biochar.
Treating the biochar with acid, bases, or metals affects the properties of biochar. It improves the formation of oxygen-containing functional groups, surface area, pore volume, and surface charge. This in turn increases the adsorption efficiency of the biochar. Modification of surface charge by impregnation with Fe salt is another strategy to improve the performance of EB.
Thus, one can modify and improve the properties of biochar by the above chemical treatments and monitor the parameters that affect the properties of biochar, and thus develop a suitable absorbent with the desired properties for adsorption of a range of pollutant molecules.
Physical methods for producing EB include processes such as ball milling, gas/steam activation, and magnetization. Ball milling is a technique that grinds biochar into fine nanoscale particles. This technique is widely used to increase the surface area, porosity, and acidic functional groups on the surface of biochar.
The modified biochar obtained by this method exhibits increased absorption capacity due to the increased surface area and porosity. The electrostatic interaction and surface complexation with the pollutants are favored by the increase in acidic functional group on the surface of biochar.
Gas/steam activation produces H2 and CO2 by surface oxidation, which is used to activate the surface of biochar. This biochar engineering technique is used to induce porosity formation, increase surface reactivity, increase specific surface area, and remove residues trapped due to incomplete combustion during pyrolysis. Biochar magnetization involves the introduction of transition metals (Fe, Co, Ni, etc.) and their oxides into the biochar matrix.
This is an effective strategy to solve the problem of separating powdered biochar from the environmental medium. Due to this additional advantage, magnetic biochar has been shown to be very effective in removing heavy metals and organic pollutants from an aqueous medium (wastewater). The production of magnetic biochar requires expertise, as improper handling can lead to clogging of the pores and a reduction in surface area.
EB can be produced by various chemical processes such as oxidation and synthesis of biochar-based composites. Oxidation with acids (HCl, H2SO4, and H2O2), bases (NaOH and KOH) or other oxidants (KMnO4 and Fe(III)) is used to charge the surface properties of biochar. Acid/base modification increases the availability of oxygen- and carbon-containing functional groups, optimizes surface electrostatic attraction, surface precipitation, and surface complexation.
When oxidants are used to activate biochar, the surface area and pore size distribution improve. Another technique to obtain EB is the synthesis of biochar-based composites with metal oxides, chitosan, amino groups, etc. The composites are prepared to improve the physicochemical properties of biochar and increase its efficiency in a particular application. For example, the composites of biochar and metal oxides have good electrostatic ability, good ion exchange, and good precipitation capacity, so they provide biochar better absorption properties.
Biological methods for EB synthesis mainly involve the use of microorganisms to obtain biochar with desired properties. The microorganism can form a biofilm on the inner and outer surfaces of biochar. This affects the pore distribution of biochar and improves its absorption and degradation capacity for various organic pollutants.
Biochar with active biofilm is a promising adsorbent for the removal of pharmaceuticals from wastewater systems. Studies have also shown that biochar modified by biological methods can be effectively used as a biofiltration medium in wastewater treatment.
Challenges and Future Research Direction with Regard to the Sustainable Development Goals (SDGs)
Although much research has been performed on biochar, there are some issues and challenges that need to be addressed. The economic and technical aspects of biochar need further study before it can be used on a large scale. The efficiency and mechanism of biochar as an absorbent have been extensively studied, but more attention should be paid to the desorption of pollutants. Regeneration of biochar is another area that can be further explored.
Studies on biochar are mostly conducted in the laboratory and in the presence of only one pollutant. Therefore, absorption by biochar in the presence of coexisting analyte should be conducted and studied in the future.
Biochar is reportedly a sustainable solution to the prevalent problem of water pollution. Biochar is derived from biomass/waste and has been shown to be a potential adsorbent for heavy metals, dyes, organic molecules, oil, etc.
As one can see there are many, many uses for BioChar. If The Thai Farmers could stop burning their Rice fields after Harvest, Air Quality would be increased, Soil Quality would be increased, (yes Carbonized Rice Hulls are good, yet this is not BioChar…) and through the use of BioChar we would be helping our environment, Tourism and more! Just like Hemp!!